By Sarah Diregger
Imagine you’re lying on your back, looking up at the sparkling stars scattered across the seemingly endless sky. The full moon drenches the nightly landscape into shades of grey. You’re enjoying the moment but a scientific thought snakes its way into your consciousness and asks, “Why do we never see a full moon during the daytime?” To help answer this question, I conducted an experiment. You’ll need:
- A flashlight
- 2 balls (preferably in two different sizes)
- An even surface
Now, you must set the two balls on the surface at a distance of approximately 10 – 20 cm. Now hold the flashlight, which represents the sun, about a meter from the two balls. I suggest using different-sized balls. The smaller one is the Moon and the larger one Earth.
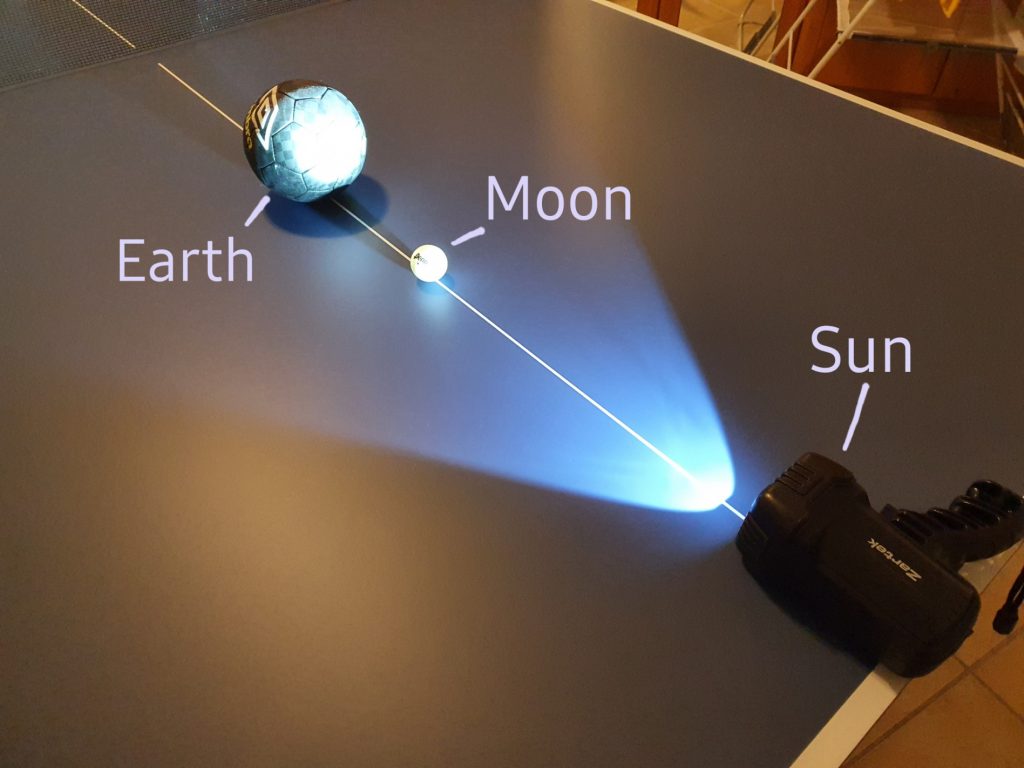
We all know that the Earth rotates around the Sun, and the Moon orbits the Earth. It’s also important to know that the Earth rotates around itself. One rotation of the Earth around itself takes 24 hours, and one rotation of the Moon around Earth takes 27,3 days.
Moving our “Moon” around the “Earth” in the experiment, we can see that depending on the position of the Moon, it’s seen from a different time of day. When the Moon is between Earth and Sun, it’s called a new moon. That means, from the point of view of Earth, you can’t see it at all.
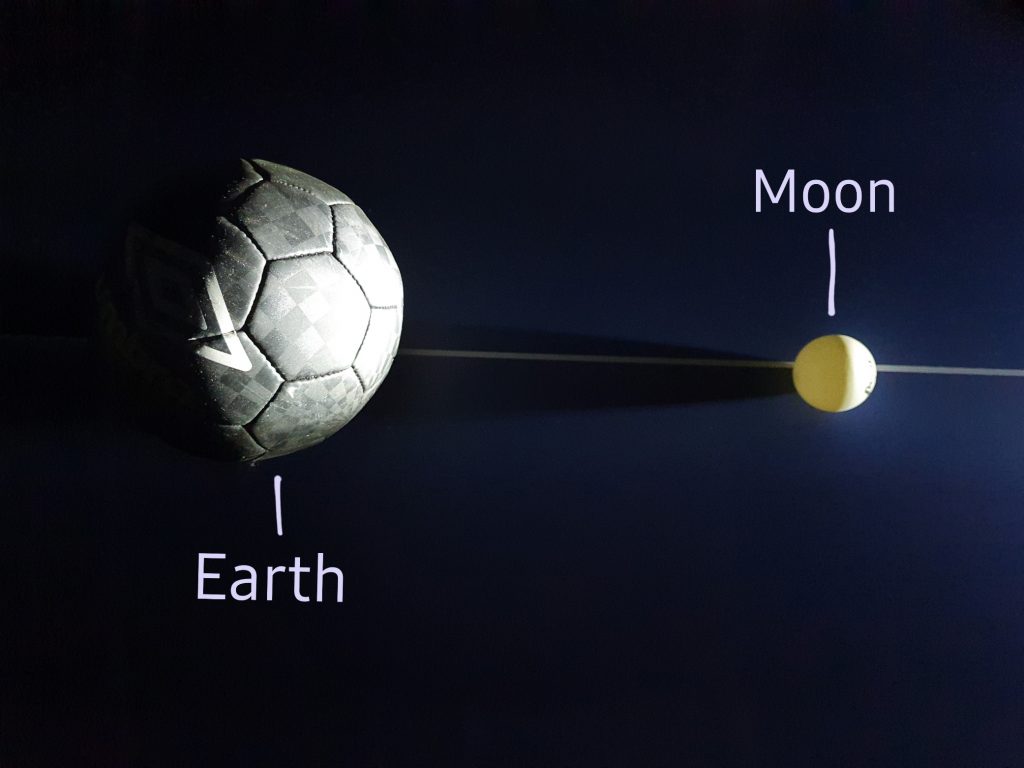
To imitate the rotation of the Moon, move it about 45° counterclockwise. You will see that from the vantage point of the Earth, a little light appears on the right side of the Moon. This waxing crescent, as we call it, can be seen at its highest position in the afternoon.
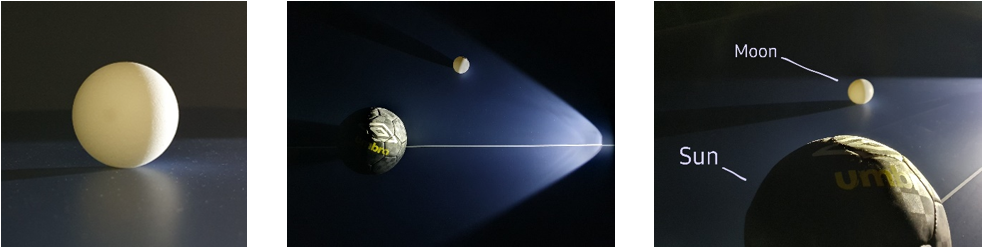
When you continue this process and move the Moon 45° counterclockwise each time, the following results can be observed:
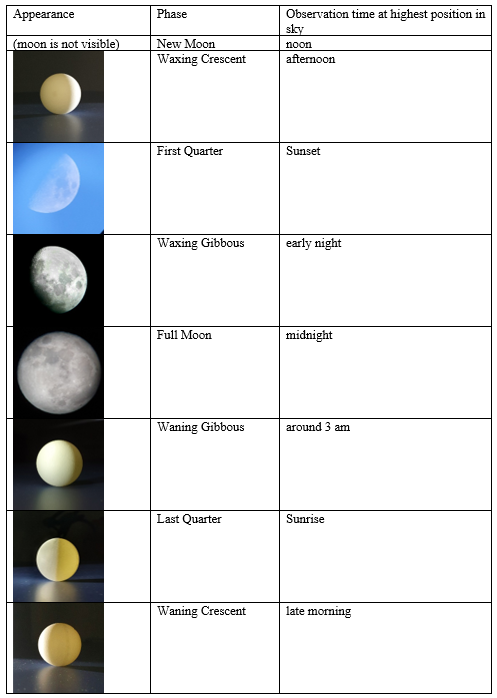
Some of the pictures above depict the real Moon and the others show our “Moon” model in the experiment.
Fun Fact:
From Earth, we always see the same side of the Moon. This is called “Tidal Locking”. It occurs when the rotation around the own axis and the rotation around another body take the same time.
Due to the position of the Moon and the Sun, we can only see a certain Moon phase in the center of the sky at a specific time of day. It’s very important to note the position of the Sun in all of this because it indicates which part of the Moon is illuminated and when we can observe this amount of illumination. That’s why we never see a full moon during the day.
Sources:
Byrd, Deborah: Moon Phases. Top 4 keys to understanding moon phases. https://earthsky.org/moon-phases/understandingmoonphases/#:~:text=As%20seen%20from%20the%20north,Not%20to%20scale. (last access: 21.03.2022)
Anon.: Lunar Phases and Eclipses. Earth’s Moon. https://solarsystem.nasa.gov/moons/earths-moon/lunar-phases-and-eclipses/#:~:text=These%20eight%20phases%20are%2C%20in,third%20quarter%20and%20waning%20crescent. (last access: 21.03.2022)
Anon.: Moon Phases. Moon in Motion. https://moon.nasa.gov/moon-in-motion/moon-phases/
Gunn, Alastair: Space. What is tidal locking?. https://www.sciencefocus.com/space/what-is-tidal-locking/ (last access: 21.03.2022)
fotocredit: © by Sarah Diregger