written by Michael Himmelbauer
By using a common shoebox, enjoyable music with a high sound quality can be played. What sounds to be unimaginable, can be realized with some components you may have at home, but can also be found in the physics room:
- an amplifier (the older the better)
- two cables
- a magnet (the stronger the better)
- a coil (or a long piece of wire and a cylinder (for example from toilet paper))
- the star of the experiment: a shoebox (without the cover)
- a device with a plug to play music
- and of course: enjoyable music (for instance on your mobile phone)
First of all, you have to glue the coil (or the wire wrapped around the cylinder) onto the bottom of the shoebox. Furthermore, the cables have to be plugged into the amplifier (take care that you use the correct ports) and have to be connected to both of the ends of the coil (no matter whether it is bought or a self-made one) as shown in the pictures:
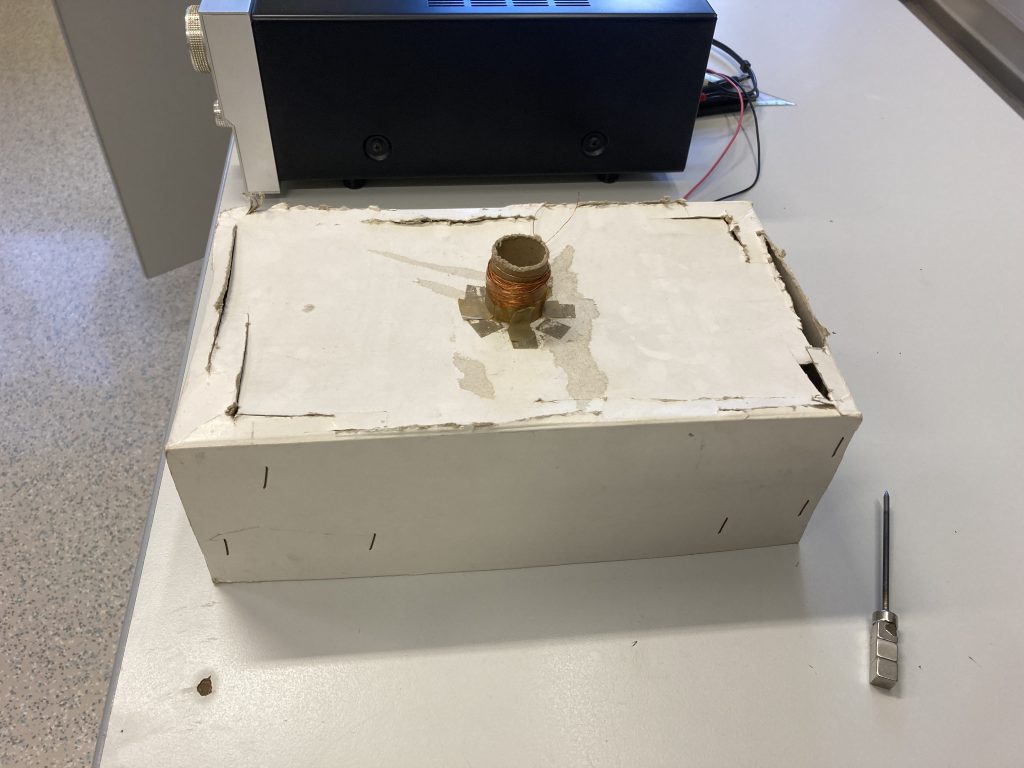
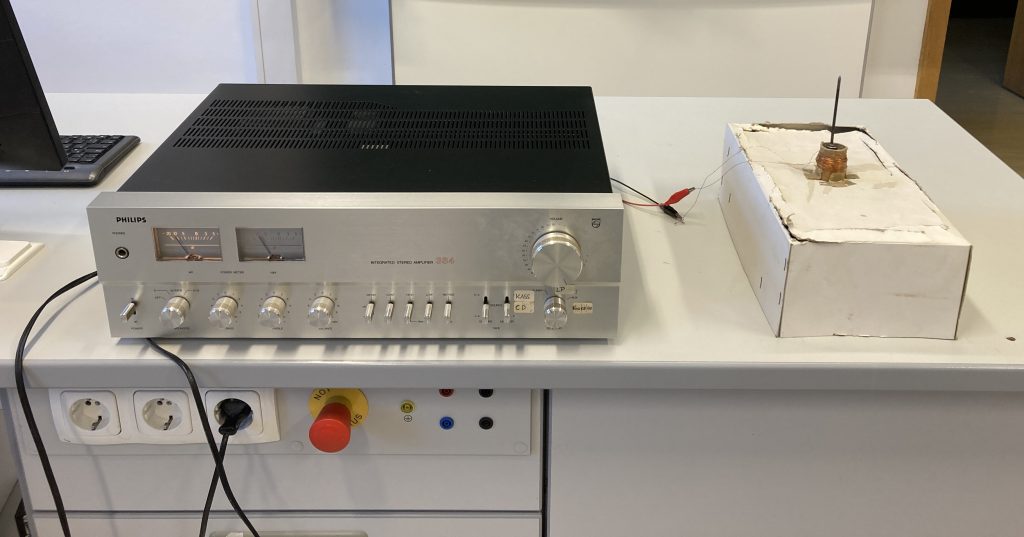
Moreover, you have to select a relaxing song to play (due to the wide range provided on the internet, this could be the most difficult part of the experiment). In order to broadcast the music, you have to connect your mobile phone, computer or MP3-player to the amplifier (either via another cable or wireless). As soon as you have started the song, place the magnet in the middle of the coil as shown in the picture:
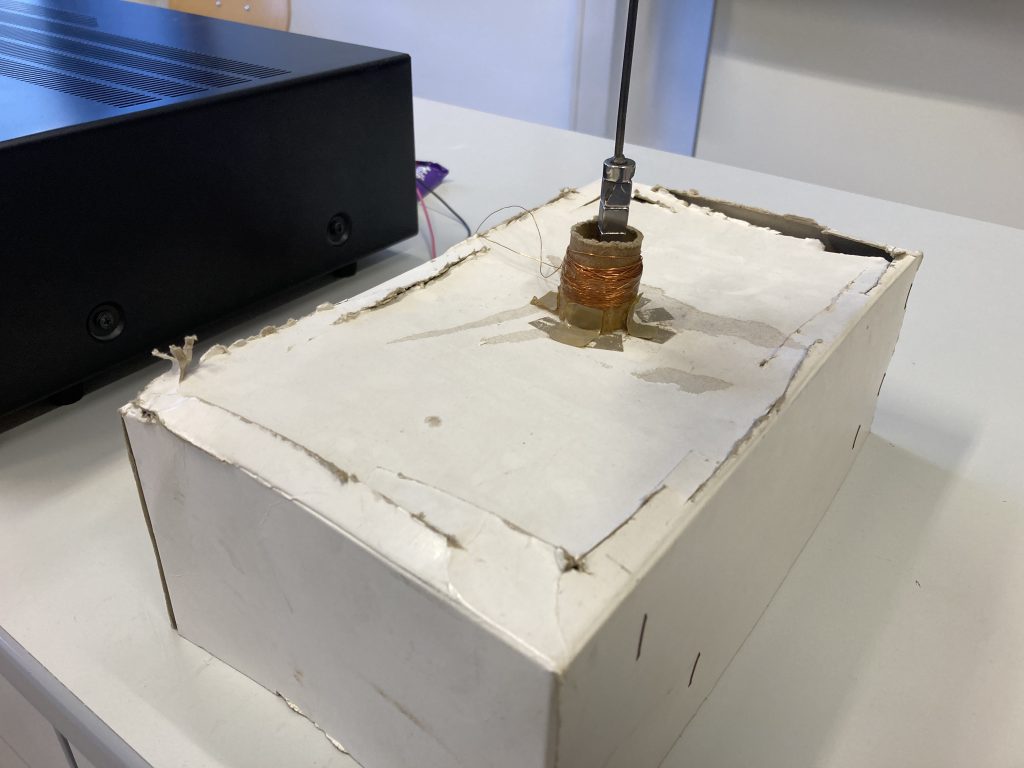
In case you read the instructions carefully and the cable connection works, you may be speechless at that point as you can hear your favorite song without using a classical speaker (or headphones).
But why’s that? Why can music be played without using speakers?
Admittedly, the shoebox works as a speaker as the mantle of the cuboid replaces the membrane.
In order to understand that phenomenon, we need to take a look at an electro-magnetic force named by the physicist Hendrik Antoon Lorentz. It occurs as soon as a current flows through a conductor. Its direction has an angle of 90 degrees to the direction of the flow and the magnetic field.
And as the amplifier dispenses alternating current (AC), the direction of the flow inside the coil changes constantly and that is why the direction of the Lorentz force changes, too. Subsequently, as the magnet remains at the same place, the coil swings back and forth with the frequency of the flow (that the amplifier dispenses). As a result of that (the coil is sticked onto the bottom of the shoebox), the membrane swings with the same frequency. Moreover, the swinging membrane spreads waves into the air that get to our ears where they are converted into an electric signal that is forwarded to the brain (ask a biologist if you’ve got any questions concerning the processes inside the human body).
To convey the theory more easily, I made a drawing that outlines the effects of the currency to the coil and depicts the formation of the acoustic waves:
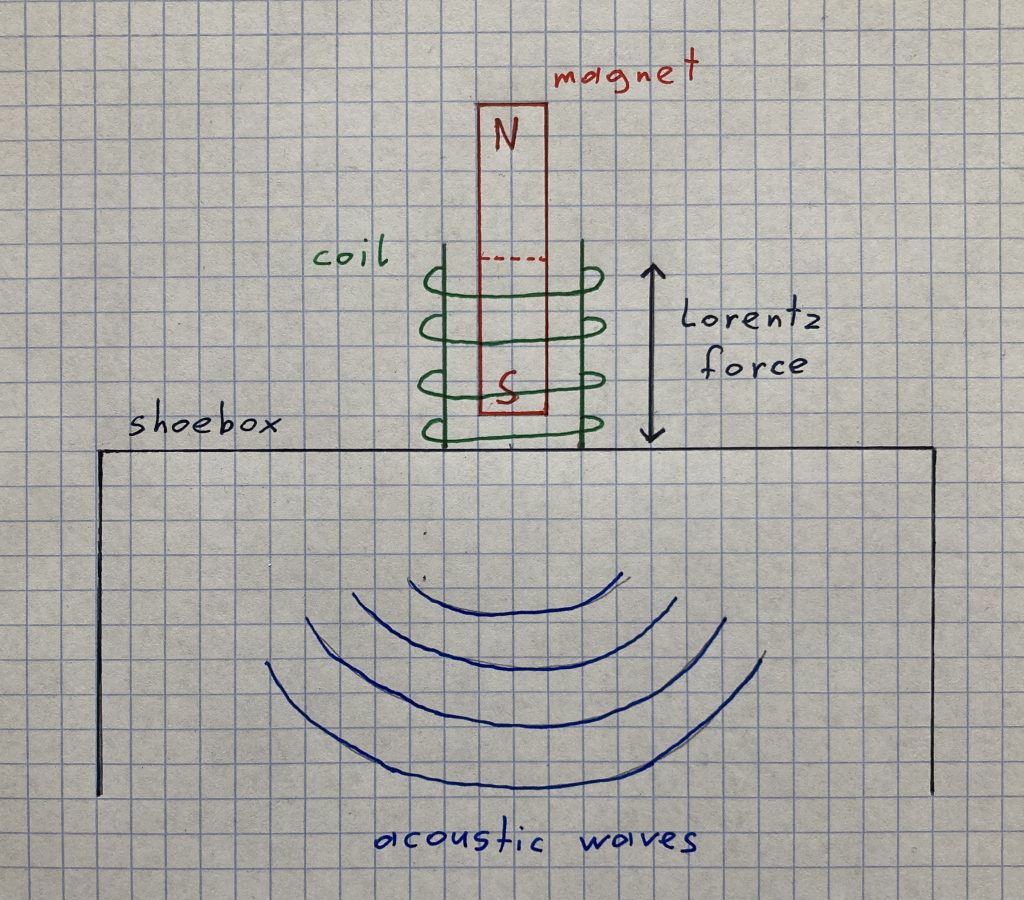
In case you are curious, you could also use the speaker for playing spoken records (for instance a speech from a well-known politician or the latest news program). While listening to the wisely chosen words from a famous person, you may be amazed by the high quality and the clear sound.
Another usage of the self-built speaker could be its opposite, a microphone. When recording waves, the Lorentz force is considered to move the electrons inside the wire and that is the reason why an electric voltage is induced (to try that, you could conduct an experiment on your own that is similar to the described one).
To sum up, it can be mentioned that with the help of a shoebox and some other components, but without a speaker, music can be played and quite some things can be found out about the characteristics of the acoustic waves that enable us to understand each other properly.
source:
Putz, Bruno; Jahn, Brigitte: Faszination Physik 7 bis 8. Lehrplan 2018. Linz: Veritas 2019, p. 13
fotocredit: (c) by Michael Himmelbauer